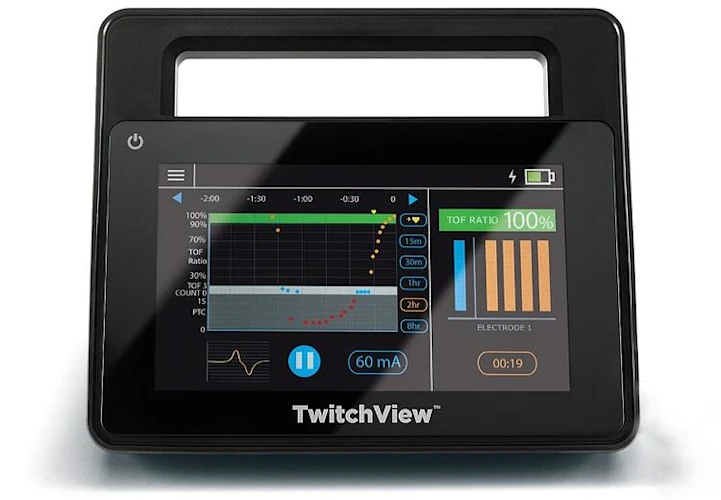
With the 2023 ASA practice guidelines for neuromuscular blockade recommending routine use of quantitative neuromuscular monitors, anesthesia departments are looking to evaluate and compare available technologies. Commercially available since the 1960s, several generations of quantitative monitors have been used in research and clinical practice. The following post reviews key features of older as well as modern quantitative monitors.
Quantitative TOF monitors are essential to implementing the 2023 American Society of Anesthesiologists Practice Guidelines for Monitoring and Antagonism of Neuromuscular Blockade in clinical practice. The guidelines were delayed partly because experts were hesitant to recommend routine use of first-generation quantitative monitors—any monitor released prior to the late 2010’s. They understood, through their own experience, that introducing flawed technology made changing practice nearly impossible. As stated in the 2018 Consensus statement on Perioperative Use of Neuromuscular Monitoring and reinforced in the 2023 ASA Practice Guidelines:
“A major limitation to widespread adoption of objective neuromuscular monitors is not their cost, but their ease of use. New monitors that will not be affected by patient hand positioning, that are self-calibrating, that are reliable, that are easy to set up, and that produce repeatable responses are needed” [1].
“While the quantitative assessment of blockade and the recognized value of the train-of-four ratio has existed for over 50 years, it has not gained widespread clinical use, largely because of the limitations of the measurement technology. Some monitors were complex to use, had poor user interfaces, or required startup/calibration times that are inconsistent with a busy clinical schedule. Some were limited in when they can be used (e.g., if the thumb cannot move, methods dependent on measuring the acceleration or strength of such movement are inaccurate). Fortunately, this situation is gradually changing with the recent introduction of substantially improved quantitative technology” [2].
Figure 1: A mechanomyograph as shown in a 1976 Anesthesiology article [5].
So, what’s different about this new technology? Fundamentally, all quantitative monitors measure muscle contraction after direct nerve stimulation. Several different modalities have been employed: acceleromyography (AMG), electromyography (EMG), kinemyography (KMG), mechanomyography (MMG), phonomyography (PMG) and compressomyography (CMG). MMG is widely acknowledged as the reference gold-standard, and MMG-based TOF ratios were used to determine the threshold for the adequate level of recovery, i.e., a TOF Ratio > 90% prior to tracheal extubation. As demonstrated by Eikermann, even modest levels of residual neuromuscular blockade (TOF ratios as high as 80%) may cause significant airway narrowing [3]. Thus, a quantitative neuromuscular monitor’s accuracy directly impacts the clinician’s ability to achieve recommended safety guidelines.
Figure 2: Minimum retroglossal upper airway diameter during forced inspiration (A) before neuromuscular blockade (baseline), at a steady-state TOF ratio of (B) 0.5 and (C) 0.8. Images from the volunteer show that a partial paralysis evokes an impairment of upper airway diameter increase during forced inspiration. *p < 0.05 versus baseline [3].
Because mechanomyography was cumbersome and difficult to use (see Figure 1), many first-generation quantitative devices designed for routine clinical use utilized acceleromyography. While more accessible than mechanomyography, two limitations restricted widespread adoption of acceleromyography in clinical practice: (1) Acceleromyography requires unrestricted thumb movement—difficult when surgical procedures call for tucked arms, and (2) Acceleromyography based readings are not interchangeable with mechanomyography and must be manually corrected, or “normalized” using a baseline TOF ratio determine prior to administration of neuromuscular blocking drugs:
“Although acceleromyography is widely used in research, it cannot be used interchangeably with mechanomyography and electromyography for construction of dose-response curves or for recording different pharmacodynamic variables after injection of a neuromuscular blocking agent” [4].
“Baseline (before neuromuscular blocking drug) train-of-four (TOF) ratio with acceleromyography is frequently > 1.0, and ranges up to about 1.4... a TOF ratio much > 1.0 is generally not seen with electromyography or mechanomyography”[5].
“Unless a baseline, unparalyzed train-of-four ratio is obtained, it is impossible to know what train-of-four ratio represents recovery from neuromuscular blockade in an individual patient, when acceleromyography is used” [6].
To overcome the limitations of acceleromyography (AMG), second-generation monitors (monitors introduced in the past 5 years) employ electromyography (EMG). Advances in microprocessors and electronics have made it possible to incorporate electromyography into compact, user-friendly monitors, and new single-use electrodes eliminate the unreliability of older reusable electromyography based technology. Figure 3 illustrates how advances in technology translate to improved electromyography monitor performance.
Figure 3: Real-time measurement comparison of an older electromyography monitor (top) to a second-generation electromyography monitor (bottom). New monitor accurately measures moderate level of neuromuscular blockade (TOFC 1) while the older monitor misidentifies artifact as patient response indicating erroneous patient recovery (TOFR 89%). Source: Author
However, interpreting electromyographic signals in environments with high levels of electrical noise (like the operating room) remains difficult. See Figure 4 for a comparison of two second-generation electromyography devices. One monitor provides reliable measurements from deep blockade through recovery, while the second monitor is unable to reliably measure the patient response until a significant level of recovery is reached, inhibiting the second monitor's ability to provide relevant clinical data during the actual case. To ensure a technology’s ability to isolate and measure the patient’s electromyography response accurately, experts have urged that all EMG-based technologies be clinically validated as interchangeable with mechanomyography—the reference gold-standard:
“Why, then, should we not rush to adopt any one of the several electromyographic twitch monitors that are now commercially available? The answer to this question requires some additional understanding of electromyography technology. The muscle compound action potentials that constitute the electromyogram are relatively small, especially in comparison to the electrical noise that is ubiquitous in an operating room. This poses a significant challenge for the engineer designing an electromyograph. Management of noise, and differentiation of noise from signal, are paramount. If noise is not effectively managed, it can be misinterpreted as compound action potentials. On the other hand, if noise reduction algorithms are too aggressive, the electrical signal from the electromyogram may be lost along with the noise, causing the monitor to underestimate twitches and lack sensitivity. Because of this, a commercially available electromyograph should be “validated” to determine how reliably it recognizes twitches, while not misinterpreting noise. In our opinion, the performance of commercially available twitch monitors should be compared to mechanomyography, using mechanomyography as the reference standard” [6].
Figure 4: Neuromuscular blockade of a single patient from case start (left) to case end (right). Overlapped measurement plots from two electromyography (EMG) monitoring technologies depict differing sensitivity and accuracy across deep, moderate and shallow neuromuscular blockade. TwitchView portrays expected linear recovery while EMG Monitor 2 is unable to measure post-tetanic count (PTC) and consistently misidentifies a TOF ratio as a TOF count until recovery (TOF ratio >90%) is achieved [7].
In summary, the newly released practice guidelines provide evidence-based recommendations for the utilization of quantitative neuromuscular monitors and antagonism of neuromuscular blockade. Technology advances have enabled the introduction of second-generation electromyography based quantitative monitors that overcome many of the limitations inherent in earlier devices. Newer acceleromyography based technologies continue to experience the same application and accuracy limitations as prior acceleromyography devices. Electromyography has been touted as the new gold standard for quantitative monitors; however, experts recommend that all technologies be validated against gold standard mechanomyography before being adopted into clinical practice.
Validation studies confirming the accuracy of TwitchView, an EMG-based second-generation neuromuscular blockade monitor have been published. TwitchView was confirmed interchangeable with the reference gold standard, mechanomyography from deep blockade through full recovery [8, 9]. For more on TwitchView, click 'Learn More' below.
References:
1. Naguib M, Brull SJ, Kopman AF, Hunter JM, Fülesdi B, Arkes HR, Elstein A, Todd MM, Johnson KB. Consensus Statement on Perioperative Use of Neuromuscular Monitoring. Anesth Analg. 2018 Jul;127(1):71-80. doi: 10.1213/ANE.0000000000002670. PMID: 29200077.
2. Stephan R. Thilen, Wade A. Weigel, Michael M. Todd, Richard P. Dutton, Cynthia A. Lien, Stuart A. Grant, Joseph W. Szokol, Lars I. Eriksson, Myron Yaster, Mark D. Grant, Madhulika Agarkar, Anne M. Marbella, Jaime F. Blanck, Karen B. Domino; 2023 American Society of Anesthesiologists Practice Guidelines for Monitoring and Antagonism of Neuromuscular Blockade: A Report by the American Society of Anesthesiologists Task Force on Neuromuscular Blockade. Anesthesiology 2023; 138:13–41 doi: https://doi.org/10.1097/ALN.0000000000004379
3. Eikermann M, Vogt FM, Herbstreit F, Vahid-Dastgerdi M, Zenge MO, Ochterbeck C, de Greiff A, Peters J. The predisposition to inspiratory upper airway collapse during partial neuromuscular blockade. Am J Respir Crit Care Med. 2007 Jan 1;175(1):9-15. doi: 10.1164/rccm.200512-1862OC. Epub 2006 Oct 5. PMID: 17023729.
4. Casper Claudius, Jørgen Viby-Mogensen, David S. Warner, Mark A. Warner; Acceleromyography for Use in Scientific and Clinical Practice: A Systematic Review of the Evidence. Anesthesiology 2008; 108:1117–1140 doi: https://doi.org/10.1097/ALN.0b013e318173f62f
5. Bowdle A, Jelacic S. Progress towards a standard of quantitative twitch monitoring. Anaesthesia. 2020 Sep;75(9):1133-1135. doi: 10.1111/anae.15009. Epub 2020 May 15. PMID: 32412668; PMCID: PMC7496266.
6. Andrew Bowdle, Kelly Michaelsen; Quantitative Twitch Monitoring: What Works Best and How Do We Know?. Anesthesiology 2021; 135:558–561 doi: https://doi.org/10.1097/ALN.0000000000003950
7. Bussey L, Jelacic S, Togashi K, Bowdle A. A Pilot Study of Train-of-Four and Post-Tetanic Count Monitoring with the TetraGraph Electromyograph Compared to the TwitchView Monitor Electromyograph.medRxiv 2021.03.19.21249475; doi: https://doi.org/10.1101/2021.03.19.21249475
8. Bowdle A, Bussey L, Michaelsen K, et al. A comparison of a prototype electromyograph vs. a mechanomyograph and an acceleromygraph for assessment of neuromuscular blockade. Anesthesia. 2020;75(2):187-195.
9. Bowdle A, Bussey L, Michaelsen K. Counting train-of-four twitch response: comparison of palpation to mechanomyography, acceleromyography, and electromyography. Br J Anaesth. 2020;124(6):712-717.